A Comprehensive Guide to Speed Breeding (with Examples)
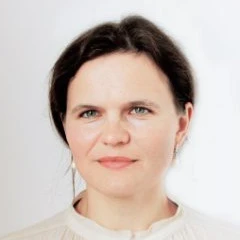
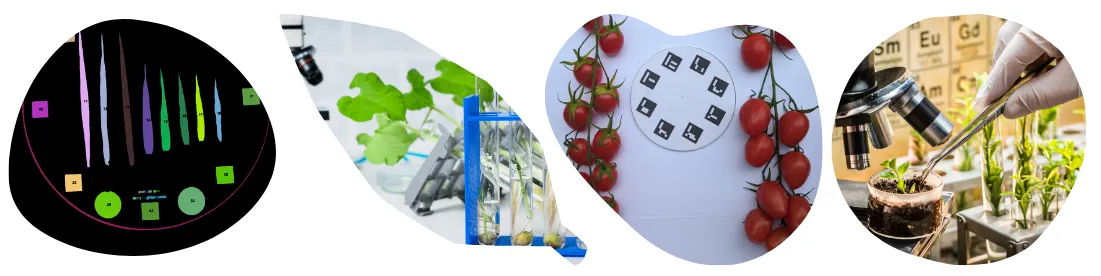
1. Historical Context of Speed Breeding
Origins and Early Development
Speed breeding, a revolutionary technique in plant science, has its roots in space exploration. The concept was initially developed by NASA in the 1980s as part of their Controlled Ecological Life Support Systems (CELSS) program. The primary goal was to find efficient ways to grow crops in space, where resources are limited and time is of the essence.
NASA’s Contribution
- 1980s: NASA scientists begin experimenting with accelerated plant growth cycles.
- 1992: First successful implementation of extended photoperiods to speed up wheat breeding.
Dr. Gary Stutte, a principal investigator for NASA, played a crucial role in these early experiments. His team demonstrated that by manipulating light cycles, they could significantly reduce the time between wheat generations.
Transition to Terrestrial Applications
While the initial focus was on space agriculture, researchers quickly recognized the potential for terrestrial applications.
Key Milestones
- Late 1990s: Australian scientists, inspired by NASA’s work, begin adapting the technique for Earth-based crop improvement.
- 2001: Dr. Lee Hickey and his team at the University of Queensland start developing speed breeding protocols for various crops.
- 2007: First published results demonstrating the successful application of speed breeding in wheat.
Achievements of speed breeding. Source - Nischay Chaudhary & Rubby Sandhu, 2024
Evolution and Refinement
The technique has undergone significant refinement since its inception:
- 2010-2015: Development of optimized light spectra and intensities for different crop species.
- 2017: Publication of a landmark paper in Nature Plants, detailing a standardized speed breeding protocol.
- 2018-present: Integration with other breeding technologies like genomic selection and CRISPR gene editing.
Global Adoption and Impact
Speed breeding has gained traction worldwide, with research institutions and companies adopting the technique:
- Europe: John Innes Centre in the UK becomes a hub for speed breeding research.
- Asia: IRRI (International Rice Research Institute) adapts the technique for rice breeding.
- Africa: ICRISAT incorporates speed breeding in its legume improvement programs.
Current State and Future Outlook
Today, speed breeding is recognized as a valuable tool in addressing global food security challenges, especially in the face of climate change. Its ability to accelerate crop development cycles has made it an integral part of many breeding programs worldwide.
As we move forward, the technique continues to evolve, with ongoing research focusing on:
- Optimizing protocols for a wider range of crop species
- Reducing energy costs and improving sustainability
- Integrating artificial intelligence for more efficient breeding decisions
The historical journey of speed breeding from a space-focused experiment to a widely adopted agricultural technique underscores its importance in modern plant science and its potential to revolutionize crop improvement strategies for years to come.
2. Theoretical Foundations of Speed Breeding
Plant Physiology Principles
Speed breeding is grounded in fundamental principles of plant physiology, particularly those related to photoperiodism and plant growth cycles.
Photoperiodism
- Definition: The physiological reaction of organisms to the length of day or night.
- Relevance: Manipulating light cycles can influence flowering time and accelerate generation turnover.
Key Physiological Processes
Photosynthesis
- Extended light periods increase daily photosynthetic accumulation.
- Optimized light spectra enhance photosynthetic efficiency.
Role of phytohormones in heat tolerance in soybean. Hormones enhance seed germination, stem elongation, and enhanced photosynthetic pigments. Source: Jianing et al., 2022
Hormonal Regulation
- Light influences plant hormone production (e.g., gibberellins, auxins).
- Altered hormone levels can accelerate growth and development.
Circadian Rhythms
- Extended photoperiods can modify plants' internal clocks.
- Understanding circadian regulation is crucial for optimizing speed breeding protocols.
Genetic Considerations
Speed breeding interacts with and influences various genetic aspects of plant development.
Genetic Factors in Accelerated Breeding
Flowering Time Genes
- Genes like FT (Flowering Locus T) play crucial roles in determining flowering time.
- Speed breeding can alter the expression of these genes.
Vernalization Requirements
- Some crops require a cold period to flower.
- Speed breeding protocols must account for or bypass these requirements.
Photoperiod Sensitivity
- Varieties with reduced photoperiod sensitivity are often more amenable to speed breeding.
Genetic Stability and Variation
- Mutation Rates: Consideration of potential increases in mutation rates under accelerated growth conditions.
- Epigenetic Changes: Evaluation of possible epigenetic modifications due to altered environmental conditions.
Physiological Stress Management
Speed breeding can induce various stresses in plants, which must be understood and managed.
Heat Stress
- Extended light periods can increase ambient temperatures.
- Understanding heat shock responses is crucial for protocol optimization.
Oxidative Stress
- Prolonged light exposure may increase reactive oxygen species production.
- Antioxidant systems play a key role in plant resilience under speed breeding conditions.
Nutrient Stress
- Accelerated growth can lead to rapid nutrient depletion.
- Optimized nutrient delivery systems are essential for successful speed breeding.
Theoretical Models
Several models have been developed to understand and predict plant responses in speed breeding environments.
Crop Growth Models
- Integration of speed breeding parameters into existing crop models.
- Development of new models specific to accelerated growth conditions.
Gene Network Models
- Mapping of gene interactions under speed breeding conditions.
- Prediction of genetic outcomes in accelerated breeding programs.
Interdisciplinary Connections
Speed breeding draws from and contributes to various scientific disciplines:
- Chronobiology: Understanding of biological rhythms in plants.
- Biophysics: Study of light-plant interactions at the molecular level.
- Computational Biology: Modeling and predicting outcomes of speed breeding protocols.
Understanding these theoretical foundations is crucial for optimizing speed breeding protocols, interpreting results, and pushing the boundaries of what’s possible in accelerated crop improvement. As research continues, these foundations are likely to evolve, potentially opening new avenues for enhancing the efficiency and effectiveness of speed breeding techniques.
Important crop growth stages and different approach to reduce their duration. In light colour shown growth stages and in deep colour shown different approach to reduce growth stage duration. Source: Imam et al., 2024
3. Detailed Methodology of Speed Breeding
This chapter provides a comprehensive, step-by-step guide to implementing speed breeding protocols.
3.1 Environmental Control Setup
3.1.1 Growth Chamber Specifications
- Temperature range: 22°C ± 3°C
- Relative humidity: 60-70%
- CO2 concentration: 400-450 ppm
3.1.2 Lighting System
- Light intensity: 400-600 μmol m⁻² s⁻¹ (PAR)
- Photoperiod: 22 hours light / 2 hours dark
- Light spectrum: Full spectrum LED with enhanced blue and red wavelengths
3.2 Plant Growth Media and Nutrition
3.2.1 Soil Mixture
- 70% peat moss, 20% vermiculite, 10% perlite
- pH adjusted to 6.0-6.5
3.2.2 Nutrient Solution
- Modified Hoagland’s solution
- EC (Electrical Conductivity): 1.5-2.0 mS/cm
- Application: Daily fertigation or automated drip system
3.3 Speed Breeding Protocol
3.3.1 Seed Preparation
- Surface sterilization with 1% sodium hypochlorite solution
- Pre-germination on moist filter paper for 24-48 hours
3.3.2 Planting
- Transfer pre-germinated seeds to growth media
- Maintain plant density at 100-150 plants/m²
3.3.3 Growth Phases Management
- Vegetative phase: 14-21 days
- Reproductive phase: 28-35 days
- Seed maturation: 14-21 days
3.3.4 Pollination Techniques
- Self-pollinating crops: Gentle shaking of plants to promote pollen dispersal
- Cross-pollinating crops: Manual cross-pollination using fine brushes
3.3.5 Seed Harvesting
- Monitor seed moisture content (target: 15-20%)
- Hand-harvest mature seeds
- Rapid drying at 35°C for 24-48 hours
3.4 Data Collection and Monitoring
3.4.1 Growth Parameters
- Plant height: Measure every 7 days
- Leaf area: Use portable leaf area meter bi-weekly
- Biomass: Fresh and dry weight at key growth stages
3.4.2 Reproductive Development
- Days to flowering
- Number of flowers/inflorescences
- Seed set rate
3.4.3 Yield Components
- Number of seeds per plant
- Thousand grain weight
- Total yield per plant/unit area
3.5 Quality Control Measures
3.5.1 Genetic Purity
- Molecular marker analysis for key traits
- Phenotypic screening for off-types
3.5.2 Seed Quality Assessment
- Germination tests: Standard and accelerated aging
- Vigor tests: Electrical conductivity and tetrazolium
3.6 Troubleshooting Common Issues
3.6.1 Plant Stress Symptoms
- Leaf chlorosis: Adjust nutrient solution or light intensity
- Stunted growth: Check for root health and adjust environmental parameters
3.6.2 Poor Seed Set
- Increase air circulation to promote pollen dispersal
- Adjust temperature during flowering (species-specific)
3.6.3 Pest and Disease Management
- Regular monitoring for early detection
- Integrated pest management strategies adapted for controlled environments
3.7 Safety Considerations
3.7.1 Personal Protective Equipment
- Eye protection for high-intensity lighting
- Respiratory protection when handling fine soil particles or chemicals
3.7.2 Electrical Safety
- Regular inspection of electrical components
- Proper grounding of all equipment
3.7.3 Chemical Safety
- Proper storage and handling of fertilizers and pesticides
- Adherence to local regulations for chemical use and disposal
By following this detailed methodology, researchers and breeders can establish and maintain effective speed breeding programs. It’s important to note that these protocols may require adjustment based on specific crop requirements and research objectives.
Certainly. Here’s the revised version:
Chapter 4: Case Studies and Future Directions in Speed Breeding
4.1 Introduction to Advanced Speed Breeding Techniques
Speed breeding has evolved significantly from its initial concept to incorporate cutting-edge technologies. As one researcher playfully noted, “Speed breeding is like speed dating 😉 but for plant genes, in controlled environmental conditions and within special scientific programs.” This analogy aptly captures the essence of the accelerated, controlled nature of the process, highlighting how quickly plant genetics can be advanced in optimized conditions.
4.2 Case Studies in Major Crops
4.2.1 Time Savings Across Various Crops
A comprehensive study from Lovely Professional University in India provided valuable insights into the application of speed breeding across multiple crop species. The researchers found that speed breeding can achieve 4-6 generations per year in various crops, compared to 1-2 generations using conventional methods. This significant increase in generational turnover has profound implications for accelerating crop improvement programs.
Timeline comparison of speed breeding with other breeding techniques. Source: Nischay Chaudhary & Rubby Sandhu, 2024
For wheat and barley, maintaining temperatures around 20-22°C allowed for up to eight generations per year, a remarkable improvement over traditional field-based breeding. In soybean studies, using a 10-hour photoperiod with specific light conditions resulted in plants maturing in just 77 days, enabling five generations per year. This rapid cycling through generations allows breeders to quickly incorporate and test new traits.
Speed breeding coupled with modern breeding techniques. Source: Nischay Chaudhary & Rubby Sandhu, 2024
Rice breeding also saw significant advancements, with optimized seedling tray conditions and thinning processes achieving a germination rate of 95-100%. Perhaps most impressively, when coupled with genomic selection, speed breeding reduced the wheat breeding cycle from 3-7 years to just 1-2 years, dramatically shortening the time to develop new varieties.
Demonstration of speed breeding in controlled environment (I), glasshouse (II) and homemade low-cost growth room (III) applied on wheat by Watson et al. Source: Nischay Chaudhary & Rubby Sandhu, 2024
4.2.2 Comprehensive Crop Studies
Another consortium of Indian agriscience research institutions conducted a wide-ranging review of speed breeding applications across various crop categories. Their work encompassed cereals, legumes, vegetables and other horticultural crops, oilseed crops, and fibre crops. This broad approach demonstrated the versatility of speed breeding techniques and their potential to revolutionize breeding programs across the agricultural spectrum.
Visual representation of different breeding methods for varietal development (Each colour represent different steps of varietal development); Black arrow - single generation, field condition; pink arrow - single generation, speed breeding. Photo credit: Imam et al., 2024
4.3 Integration of AI and Advanced Technologies
The future of speed breeding lies in its integration with other advanced technologies. CRISPR-based gene editing offers the potential for precise genetic modifications, allowing breeders to target specific traits with unprecedented accuracy. High-throughput phenotyping technologies enable rapid assessment of plant traits, providing crucial data to inform breeding decisions.
Genomic selection, when combined with speed breeding, allows for more accurate predictions of breeding outcomes, further accelerating the development of improved varieties. Perhaps most excitingly, AI-driven control systems are being developed to optimize environmental conditions for multiple generations and diverse crops simultaneously, pushing the boundaries of what’s possible in controlled environment agriculture.
4.4 AI vs. Conventional Breeding: A Comparative Study
A comparative study published in February 2024 by Krishna Kumar Rai from Banaras Hindu University highlighted the advantages of AI in plant breeding. The research underscored the limitations of conventional breeding techniques, which are often time-consuming and labor-intensive. In contrast, AI applications offer real-time monitoring of plant responses to environmental changes, exploration of complex plant mechanisms, and analysis of OMICS datasets.
These AI-driven approaches are proving instrumental in developing high-yield, adaptable crop plants more efficiently. By leveraging machine learning algorithms and big data analytics, researchers can identify subtle patterns and relationships that might be missed by human observers, leading to more informed breeding decisions and ultimately more resilient and productive crop varieties.
An overview of the potential application of artificial intelligence in augmenting plant breeding technology for easy, precise, and early prediction of genotypes/parental combinations for varietal development. Photo credit: Krishna Kumar Rai, 2024
4.5 Future Directions and Challenges
As speed breeding continues to evolve, several key areas for future development have emerged. Further integration of AI is a primary focus, with researchers working to develop more sophisticated models for predicting optimal breeding conditions and outcomes. These models promise to take much of the guesswork out of breeding programs, allowing for more targeted and efficient variety development.
Sustainability concerns are also at the forefront, as researchers grapple with addressing the energy consumption of controlled environments used in speed breeding. Innovations in LED lighting and climate control systems are helping to mitigate these concerns, but more work remains to be done.
Scaling up successful speed breeding protocols from laboratory to large-scale agricultural applications presents another significant challenge. This transition will require careful planning and investment in infrastructure to ensure that the benefits of speed breeding can be realized on a commercial scale.
Maintaining genetic diversity is a critical consideration as breeding cycles accelerate. Researchers must be vigilant to ensure that the push for rapid improvement doesn’t lead to a narrowing of the genetic base, which could leave crops vulnerable to future stresses.
Finally, the development of appropriate regulatory frameworks for crops developed through speed breeding, especially when combined with gene editing, will be crucial for public acceptance and commercial viability of these new varieties.
4.6 Conclusion
Speed breeding, enhanced by AI and other advanced technologies, represents a significant leap forward in crop improvement. The case studies from India and elsewhere demonstrate its potential to dramatically reduce breeding cycles and accelerate the development of crops adapted to changing environmental conditions. As we move forward, addressing challenges related to sustainability, genetic diversity, and regulatory compliance will be crucial to fully realizing the benefits of these advanced breeding techniques. The future of agriculture looks brighter and more efficient, thanks to the innovative approaches of speed breeding and its integration with cutting-edge technologies.
In conclusion, these studies demonstrate the significant progress being made in stress breeding across various crops. By combining traditional breeding techniques with cutting-edge technologies and data analysis, researchers are paving the way for more resilient and productive crops in the face of climate change.